Bacterial microcompartments are giant, polyhedrally shaped
structures found within many bacteria. They consist of a thin
outer protein shell assembled from a few thousand protein
subunits -- in a fashion reminiscent of a viral capsid -- encapsulating
a series of sequentially-acting enzymes. They function as
simple protein-based metabolic organelles by sequestering key
pathways within the cell. The carboxysome is the founding
member; it encapsulates the enzymes carbonic anhydrase and
RuBisCO in cyanabacteria and some chemoautotrophs in order
to enhance CO2 fixation. Other types of microcompartments
have been studied that metabolize two and three carbon compunds
such as ethanolamine and 1,2-propanediol in various enteric
bacteria. Those microcompartments serve to retain chemically
reactive and/or volatile pathway intermediates like acetaldehyde
and propionaldehyde so they can be converted to less reactive
compounds before being released into the cytosol.
We have determined crystal structures of many of the shell proteins that compose
various microcompartments, including from the carboxysome, the Pdu (propanediol utilization),
and the Eut (ethanolamine) shells. These have been extremely helpful in
illuminating form and function. From these studies we understand that
the main shell proteins (known as BMC proteins) assemble as hexamers, which then
further assemble side-by-side in a molecular sheet that forms the flat facets
of the intact shell. In our models, special pentameric shell proteins are
postulated to form the vertices of the icosahedral-like shell. These
valuable structural findings are the result of efforts by numerous graduate
students, postodocs and researchers in the laboratory over the years,
beginning with Kerfeld et al. in 2005.
Each of the hexamers bears a narrow central pore.
These pores are presumed to be the routes by which substrates and products
-- and likely cofactors for some of the enclosed enzymatic reactions -- cross
into and out of the microcompartment. Some of the shell proteins undergo conformational
changes between open and closed forms, providing a clue about how transport
might be controlled. We have also determined that certain special shell proteins
bind an iron-sulfur metal cluster at the center of their pore, we presume in
order to facilitate transport of electrons or possibly intact metal clusters;
most microcompartment types involve internal redox reactions as well
as internal iron-sulfur enzymes. Our collaborator, Tom Bobik at Iowa State, has established
that some of the enzymes inside microcompartments are targeted to the interior surface of
the shell by special N-terminal sequence extensions. An understanding of this mechanism
has opened up prospects for engineering microcompartments with novel interior enzymes.
Our ongoing aims are: to provide futher structural data in order to establish more
complete three-dimensional models of microcompartments; to engineer microcompartments with different
properties; and to use bioinformatics methods to discover new types of compartments
in bacteria.
References:
- Yeates TO, Thompson MC, Bobik TA. (2011).
The protein shells of bacterial microcompartment organelles.
Curr. Opin. Struct. Biol.. Apr 2011. 21(2):223-31.
[Abstract]
Details are emerging on the structure and function of a remarkable class of capsid-like protein assemblies that serve as simple metabolic organelles in many bacteria. These bacterial microcompartments consist of a few thousand shell proteins, which encapsulate two or more sequentially acting enzymes in order to enhance or sequester certain metabolic pathways, particularly those involving toxic or volatile intermediates. Genomic data indicate that bacterial microcompartment shell proteins are present in a wide range of bacterial species, where they encapsulate varied reactions. Crystal structures of numerous shell proteins from distinct types of microcompartments have provided keys for understanding how the shells are assembled and how they conduct molecular transport into and out of microcompartments. The structural data emphasize a high level of mechanistic sophistication in the protein shell, and point the way for further studies on this fascinating but poorly appreciated class of subcellular structures.
- Yeates TO, Crowley CS, Tanaka S. (2010).
Bacterial microcompartment organelles: protein shell structure and evolution.
Annu Rev Biophys. 2010. 39:185-205.
[Abstract]
Some bacteria contain organelles or microcompartments consisting of a large virion-like protein shell encapsulating sequentially acting enzymes. These organized microcompartments serve to enhance or protect key metabolic pathways inside the cell. The variety of bacterial microcompartments provide diverse metabolic functions, ranging from CO(2) fixation to the degradation of small organic molecules. Yet they share an evolutionarily related shell, which is defined by a conserved protein domain that is widely distributed across the bacterial kingdom. Structural studies on a number of these bacterial microcompartment shell proteins are illuminating the architecture of the shell and highlighting its critical role in controlling molecular transport into and out of microcompartments. Current structural, evolutionary, and mechanistic ideas are discussed, along with genomic studies for exploring the function and diversity of this family of bacterial organelles.
- Tanaka S, Sawaya MR, Yeates TO. (2010).
Structure and mechanisms of a protein-based organelle in Escherichia coli.
Science. Jan 2010. 327(5961):81-4.
[Abstract]
Many bacterial cells contain proteinaceous microcompartments that act as simple organelles by sequestering specific metabolic processes involving volatile or toxic metabolites. Here we report the three-dimensional (3D) crystal structures, with resolutions between 1.65 and 2.5 angstroms, of the four homologous proteins (EutS, EutL, EutK, and EutM) that are thought to be the major shell constituents of a functionally complex ethanolamine utilization (Eut) microcompartment. The Eut microcompartment is used to sequester the metabolism of ethanolamine in bacteria such as Escherichia coli and Salmonella enterica. The four Eut shell proteins share an overall similar 3D fold, but they have distinguishing structural features that help explain the specific roles they play in the microcompartment. For example, EutL undergoes a conformational change that is probably involved in gating molecular transport through shell protein pores, whereas structural evidence suggests that EutK might bind a nucleic acid component. Together these structures give mechanistic insight into bacterial microcompartments.
- Fan C, Cheng S, Liu Y, Escobar CM, Crowley CS, Jefferson RE, Yeates TO, Bobik TA. (2010).
Short N-terminal sequences package proteins into bacterial microcompartments.
Proc. Natl. Acad. Sci. U.S.A.. Apr 2010. 107(16):7509-14.
[Abstract]
Hundreds of bacterial species produce proteinaceous microcompartments (MCPs) that act as simple organelles by confining the enzymes of metabolic pathways that have toxic or volatile intermediates. A fundamental unanswered question about bacterial MCPs is how enzymes are packaged within the protein shell that forms their outer surface. Here, we report that a short N-terminal peptide is necessary and sufficient for packaging enzymes into the lumen of an MCP involved in B(12)-dependent 1,2-propanediol utilization (Pdu MCP). Deletion of 10 or 14 amino acids from the N terminus of the propionaldehyde dehydrogenase (PduP) enzyme, which is normally found within the Pdu MCP, substantially impaired packaging, with minimal effects on its enzymatic activity. Fusion of the 18 N-terminal amino acids from PduP to GFP, GST, or maltose-binding protein resulted in their encapsulation within MCPs. Bioinformatic analyses revealed N-terminal extensions in two additional Pdu proteins and three proteins from two unrelated MCPs, suggesting that N-terminal peptides may be used to package proteins into diverse MCPs. The potential uses of MCP assembly principles in nature and in biotechnology are discussed.
- Crowley CS, Cascio D, Sawaya MR, Kopstein JS, Bobik TA, Yeates TO. (2010).
Structural insight into the mechanisms of transport across the Salmonella enterica Pdu microcompartment shell.
J. Biol. Chem.. Nov 2010. 285(48):37838-46.
[Abstract]
Bacterial microcompartments are a functionally diverse group of proteinaceous organelles that confine specific reaction pathways in the cell within a thin protein-based shell. The propanediol utilizing (Pdu) microcompartment contains the reactions for metabolizing 1,2-propanediol in certain enteric bacteria, including Salmonella. The Pdu shell is assembled from a few thousand protein subunits of several different types. Here we report the crystal structures of two key shell proteins, PduA and PduT. The crystal structures offer insights into the mechanisms of Pdu microcompartment assembly and molecular transport across the shell. PduA forms a symmetric homohexamer whose central pore appears tailored for facilitating transport of the 1,2-propanediol substrate. PduT is a novel, tandem domain shell protein that assembles as a pseudohexameric homotrimer. Its structure reveals an unexpected site for binding an [Fe-S] cluster at the center of the PduT pore. The location of a metal redox cofactor in the pore of a shell protein suggests a novel mechanism for either transferring redox equivalents across the shell or for regenerating luminal [Fe-S] clusters.
- Tanaka S, Sawaya MR, Phillips M, Yeates TO. (2009).
Insights from multiple structures of the shell proteins from the beta-carboxysome.
Protein Sci.. Jan 2009. 18(1):108-20.
[Abstract]
Carboxysomes are primitive bacterial organelles that function as a part of a carbon concentrating mechanism (CCM) under conditions where inorganic carbon is limiting. The carboxysome enhances the efficiency of cellular carbon fixation by encapsulating together carbonic anhydrase and the CO(2)-fixing enzyme ribulose-1,5-bisphosphate carboxylase/oxygenase (RuBisCO). The carboxysome has a roughly icosahedral shape with an outer shell between 800 and 1500 A in diameter, which is constructed from a few thousand small protein subunits. In the cyanobacterium Synechocystis sp. PCC 6803, the previous structure determination of two homologous shell protein subunits, CcmK2 and CcmK4, elucidated how the outer shell is formed by the tight packing of CcmK hexamers into a molecular layer. Here we describe the crystal structure of the hexameric shell protein CcmK1, along with structures of mutants of both CcmK1 and CcmK2 lacking their sometimes flexible C-terminal tails. Variations in the way hexamers pack into layers are noted, while sulfate ions bound in pores through the layer provide further support for the hypothesis that the pores serve for transport of substrates and products into and out of the carboxysome. One of the new structures provides a high-resolution (1.3 A) framework for subsequent computational studies of molecular transport through the pores. Crystal and solution studies of the C-terminal deletion mutants demonstrate the tendency of the terminal segments to participate in protein--protein interactions, thereby providing a clue as to which side of the molecular layer of hexameric shell proteins is likely to face toward the carboxysome interior.
- Beeby M, Bobik TA, Yeates TO. (2009).
Exploiting genomic patterns to discover new supramolecular protein assemblies.
Protein Sci.. Jan 2009. 18(1):69-79.
[Abstract]
Bacterial microcompartments are supramolecular protein assemblies that function as bacterial organelles by compartmentalizing particular enzymes and metabolic intermediates. The outer shells of these microcompartments are assembled from multiple paralogous structural proteins. Because the paralogs are required to assemble together, their genes are often transcribed together from the same operon, giving rise to a distinctive genomic pattern: multiple, typically small, paralogous proteins encoded in close proximity on the bacterial chromosome. To investigate the generality of this pattern in supramolecular assemblies, we employed a comparative genomics approach to search for protein families that show the same kind of genomic pattern as that exhibited by bacterial microcompartments. The results indicate that a variety of large supramolecular assemblies fit the pattern, including bacterial gas vesicles, bacterial pili, and small heat-shock protein complexes. The search also retrieved several widely distributed protein families of presently unknown function. The proteins from one of these families were characterized experimentally and found to show a behavior indicative of supramolecular assembly. We conclude that cotranscribed paralogs are a common feature of diverse supramolecular assemblies, and a useful genomic signature for discovering new kinds of large protein assemblies from genomic data.
- Dryden KA, Crowley CS, Tanaka S, Yeates TO, Yeager M. (2009).
Two-dimensional crystals of carboxysome shell proteins recapitulate the hexagonal packing of three-dimensional crystals.
Protein Sci.. Dec 2009. 18(12):2629-35.
[Abstract]
Bacterial microcompartments (BMCs) are large intracellular bodies that serve as simple organelles in many bacteria. They are proteinaceous structures composed of key enzymes encapsulated by a polyhedral protein shell. In previous studies, the organization of these large shells has been inferred from the conserved packing of the component shell proteins in two-dimensional (2D) layers within the context of three-dimensional (3D) crystals. Here, we show that well-ordered, 2D crystals of carboxysome shell proteins assemble spontaneously when His-tagged proteins bind to a monolayer of nickelated lipid molecules at an air-water interface. The molecular packing within the 2D crystals recapitulates the layered hexagonal sheets observed in 3D crystals. The results reinforce current models for the molecular design of BMC shells.
- Tanaka S, Kerfeld CA, Sawaya MR, Cai F, Heinhorst S, Cannon GC, Yeates TO. (2008).
Atomic-level models of the bacterial carboxysome shell.
Science. Feb 2008. 319(5866):1083-6.
[Abstract]
The carboxysome is a bacterial microcompartment that functions as a simple organelle by sequestering enzymes involved in carbon fixation. The carboxysome shell is roughly 800 to 1400 angstroms in diameter and is assembled from several thousand protein subunits. Previous studies have revealed the three-dimensional structures of hexameric carboxysome shell proteins, which self-assemble into molecular layers that most likely constitute the facets of the polyhedral shell. Here, we report the three-dimensional structures of two proteins of previously unknown function, CcmL and OrfA (or CsoS4A), from the two known classes of carboxysomes, at resolutions of 2.4 and 2.15 angstroms. Both proteins assemble to form pentameric structures whose size and shape are compatible with formation of vertices in an icosahedral shell. Combining these pentamers with the hexamers previously elucidated gives two plausible, preliminary atomic models for the carboxysome shell.
- Crowley CS, Sawaya MR, Bobik TA, Yeates TO. (2008).
Structure of the PduU shell protein from the Pdu microcompartment of Salmonella.
Structure. Sep 2008. 16(9):1324-32.
[Abstract]
The Pdu microcompartment is a proteinaceous, subcellular structure that serves as an organelle for the metabolism of 1,2-propanediol in Salmonella enterica. It encapsulates several related enzymes within a shell composed of a few thousand protein subunits. Recent structural studies on the carboxysome, a related microcompartment involved in CO(2) fixation, have concluded that the major shell proteins from that microcompartment form hexamers that pack into layers comprising the facets of the shell. Here we report the crystal structure of PduU, a protein from the Pdu microcompartment, representing the first structure of a shell protein from a noncarboxysome microcompartment. Though PduU is a hexamer like other characterized shell proteins, it has undergone a circular permutation leading to dramatic differences in the hexamer pore. In view of the hypothesis that microcompartment metabolites diffuse across the outer shell through these pores, the unique structure of PduU suggests the possibility of a special functional role.
- Yeates TO, Kerfeld CA, Heinhorst S, Cannon GC, Shively JM. (2008).
Protein-based organelles in bacteria: carboxysomes and related microcompartments.
Nat. Rev. Microbiol.. Sep 2008. 6(9):681-91.
[Abstract]
Many bacteria contain intracellular microcompartments with outer shells that are composed of thousands of protein subunits and interiors that are filled with functionally related enzymes. These microcompartments serve as organelles by sequestering specific metabolic pathways in bacterial cells. The carboxysome, a prototypical bacterial microcompartment that is found in cyanobacteria and some chemoautotrophs, encapsulates ribulose-l,5-bisphosphate carboxylase/oxygenase (RuBisCO) and carbonic anhydrase, and thereby enhances carbon fixation by elevating the levels of CO2 in the vicinity of RuBisCO. Evolutionarily related, but functionally distinct, microcompartments are present in diverse bacteria. Although bacterial microcompartments were first observed more than 40 years ago, a detailed understanding of how they function is only now beginning to emerge.
- Cheng S, Liu Y, Crowley CS, Yeates TO, Bobik TA. (2008).
Bacterial microcompartments: their properties and paradoxes.
Bioessays. Nov 2008. 30(11-12):1084-95.
[Abstract]
Many bacteria conditionally express proteinaceous organelles referred to here as microcompartments (Fig. 1). These microcompartments are thought to be involved in a least seven different metabolic processes and the number is growing. Microcompartments are very large and structurally sophisticated. They are usually about 100-150 nm in cross section and consist of 10,000-20,000 polypeptides of 10-20 types. Their unifying feature is a solid shell constructed from proteins having bacterial microcompartment (BMC) domains. In the examples that have been studied, the microcompartment shell encases sequentially acting metabolic enzymes that catalyze a reaction sequence having a toxic or volatile intermediate product. It is thought that the shell of the microcompartment confines such intermediates, thereby enhancing metabolic efficiency and/or protecting cytoplasmic components. Mechanistically, however, this creates a paradox. How do microcompartments allow enzyme substrates, products and cofactors to pass while confining metabolic intermediates in the absence of a selectively permeable membrane? We suggest that the answer to this paradox may have broad implications with respect to our understanding of the fundamental properties of biological protein sheets including microcompartment shells, S-layers and viral capsids.
- Tsai Y, Sawaya MR, Cannon GC, Cai F, Williams EB, Heinhorst S, Kerfeld CA, Yeates TO. (2007).
Structural analysis of CsoS1A and the protein shell of the Halothiobacillus neapolitanus carboxysome.
PLoS Biol.. Jun 2007. 5(6):e144.
[Abstract]
The carboxysome is a bacterial organelle that functions to enhance the efficiency of CO2 fixation by encapsulating the enzymes ribulose bisphosphate carboxylase/oxygenase (RuBisCO) and carbonic anhydrase. The outer shell of the carboxysome is reminiscent of a viral capsid, being constructed from many copies of a few small proteins. Here we describe the structure of the shell protein CsoS1A from the chemoautotrophic bacterium Halothiobacillus neapolitanus. The CsoS1A protein forms hexameric units that pack tightly together to form a molecular layer, which is perforated by narrow pores. Sulfate ions, soaked into crystals of CsoS1A, are observed in the pores of the molecular layer, supporting the idea that the pores could be the conduit for negatively charged metabolites such as bicarbonate, which must cross the shell. The problem of diffusion across a semiporous protein shell is discussed, with the conclusion that the shell is sufficiently porous to allow adequate transport of small molecules. The molecular layer formed by CsoS1A is similar to the recently observed layers formed by cyanobacterial carboxysome shell proteins. This similarity supports the argument that the layers observed represent the natural structure of the facets of the carboxysome shell. Insights into carboxysome function are provided by comparisons of the carboxysome shell to viral capsids, and a comparison of its pores to the pores of transmembrane protein channels.
- Yeates TO, Tsai Y, Tanaka S, Sawaya MR, Kerfeld CA. (2007).
Self-assembly in the carboxysome: a viral capsid-like protein shell in bacterial cells.
Biochem. Soc. Trans.. Jun 2007. 35(Pt 3):508-11.
[Abstract]
Many proteins self-assemble to form large supramolecular complexes. Numerous examples of these structures have been characterized, ranging from spherical viruses to tubular protein assemblies. Some new kinds of supramolecular structures are just coming to light, while it is likely there are others that have not yet been discovered. The carboxysome is a subcellular structure that has been known for more than 40 years, but whose structural and functional details are just now emerging. This giant polyhedral body is constructed as a closed shell assembled from several thousand protein subunits. Within this protein shell, the carboxysome encapsulates the CO(2)-fixing enzymes, Rubisco (ribulose-1,5-bisphosphate carboxylase/oxygenase) and carbonic anhydrase; this arrangement enhances the efficiency of cellular CO(2) fixation. The carboxysome is present in many photosynthetic and chemoautotrophic bacteria, and so plays an important role in the global carbon cycle. It also serves as the prototypical member of what appears to be a large class of primitive protein-based organelles in bacteria. A series of crystal structures is beginning to reveal the secrets of how the carboxysome is assembled and how it enhances the efficiency of CO(2) fixation. Some of the assembly principles revealed in the carboxysome are reminiscent of those seen in icosahedral viral capsids. In addition, the shell appears to be perforated by pores for metabolite transport into and out of the carboxysome, suggesting comparisons to the pores through oligomeric transmembrane proteins, which serve to transport small molecules across the membrane bilayers of cells and eukaryotic organelles.
- Sawaya MR, Cannon GC, Heinhorst S, Tanaka S, Williams EB, Yeates TO, Kerfeld CA. (2006).
The structure of beta-carbonic anhydrase from the carboxysomal shell reveals a distinct subclass with one active site for the price of two.
J. Biol. Chem.. Mar 2006. 281(11):7546-55.
[Abstract]
CsoSCA (formerly CsoS3) is a bacterial carbonic anhydrase localized in the shell of a cellular microcompartment called the carboxysome, where it converts HCO(3)(-) to CO(2) for use in carbon fixation by ribulose-bisphosphate carboxylase/oxygenase (RuBisCO). CsoSCA lacks significant sequence similarity to any of the four known classes of carbonic anhydrase (alpha, beta, gamma, or delta), and so it was initially classified as belonging to a new class, epsilon. The crystal structure of CsoSCA from Halothiobacillus neapolitanus reveals that it is actually a representative member of a new subclass of beta-carbonic anhydrases, distinguished by a lack of active site pairing. Whereas a typical beta-carbonic anhydrase maintains a pair of active sites organized within a two-fold symmetric homodimer or pair of fused, homologous domains, the two domains in CsoSCA have diverged to the point that only one domain in the pair retains a viable active site. We suggest that this defunct and somewhat diminished domain has evolved a new function, specific to its carboxysomal environment. Despite the level of sequence divergence that separates CsoSCA from the other two subclasses of beta-carbonic anhydrases, there is a remarkable level of structural similarity among active site regions, which suggests a common catalytic mechanism for the interconversion of HCO(3)(-) and CO(2). Crystal packing analysis suggests that CsoSCA exists within the carboxysome shell either as a homodimer or as extended filaments.
- Kerfeld CA, Sawaya MR, Tanaka S, Nguyen CV, Phillips M, Beeby M, Yeates TO. (2005).
Protein structures forming the shell of primitive bacterial organelles.
Science. Aug 2005. 309(5736):936-8.
[Abstract]
Bacterial microcompartments are primitive organelles composed entirely of protein subunits. Genomic sequence databases reveal the widespread occurrence of microcompartments across diverse microbes. The prototypical bacterial microcompartment is the carboxysome, a protein shell for sequestering carbon fixation reactions. We report three-dimensional crystal structures of multiple carboxysome shell proteins, revealing a hexameric unit as the basic microcompartment building block and showing how these hexamers assemble to form flat facets of the polyhedral shell. The structures suggest how molecular transport across the shell may be controlled and how structural variations might govern the assembly and architecture of these subcellular compartments.
|
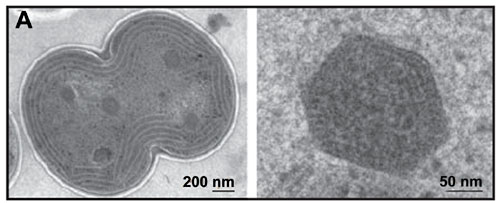
Electron micrograph of carboxysomes (courtesy of Vim Vermaas, adapted from Kerfeld, et al., 2005)
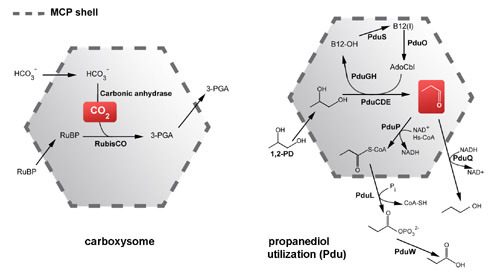
Metabolic functions of two kinds of microcompartments. (Adapted from Yeates, Crowley, & Tanaka, 2010)
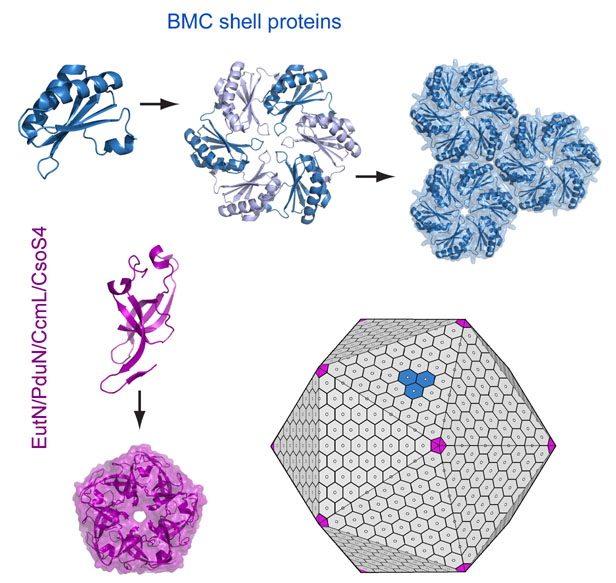
A simplified model of microcompartment assembly. (Adapted from Yeates, Thompson & Bobik, 2011)
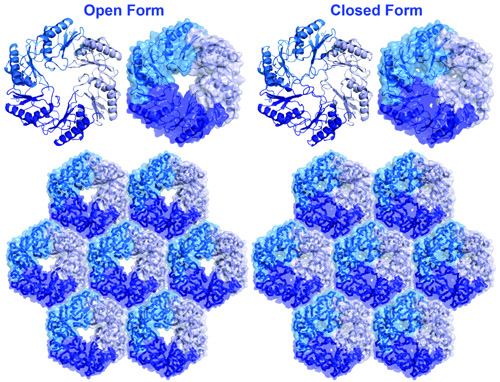
Open and closed pore forms of a BMC shell protein (EutL). (Adapted from Tanaka, et al., 2010)
|